Multiple nanoemulsions
The structural and chemical uniformity of traditional emulsions facilitates their production; however, this simplicity also limits the scope of their applications. Multiphase or ‘multiple’ emulsions have increased complexity and potentially broader utility than conventional emulsions, owing to their internalized phases, enabling chemical compartmentalization, control of active-ingredient release and complex particle templates. However, multiple-emulsion droplets prepared with conventional methods are too large for emerging applications. As a result, there has been increased research interest in the development of multiple nanoemulsions, whereby the nanoscale droplet size overcomes many of the limitations of more common, micrometre-scale multiple emulsions. Although numerous successful demonstrations of multiple-nanoemulsion production have emerged, there remain considerable challenges to improve stability, control over internal structure and characterization of multiphase nanodroplets. Overcoming these challenges provides unique opportunities to exploit the hierarchical structure, enhanced colloidal stability and multiphase chemical solubilization for applications, such as delivery vehicles, chemical sinks and particle templates, with functions that are otherwise inaccessible. In this Review, we summarize the techniques used to generate and characterize multiple nanoemulsions, theories to understand their formation and stability, and discuss current and future applications that are, or may be, enabled by their unique structures and chemistries.
This is a preview of subscription content, access via your institution
Access options
Access Nature and 54 other Nature Portfolio journals
Get Nature+, our best-value online-access subscription
cancel any time
Subscribe to this journal
Receive 12 digital issues and online access to articles
133,45 € per year
only 11,12 € per issue
Buy this article
- Purchase on SpringerLink
- Instant access to full article PDF
Prices may be subject to local taxes which are calculated during checkout
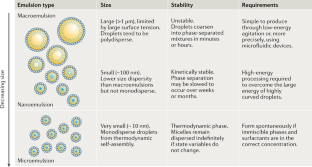
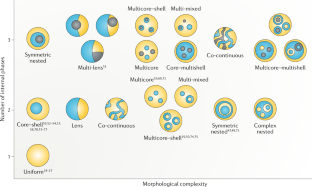
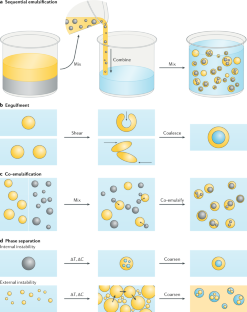
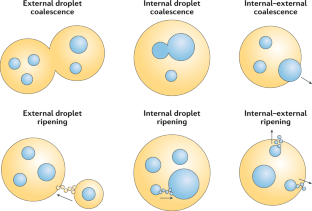
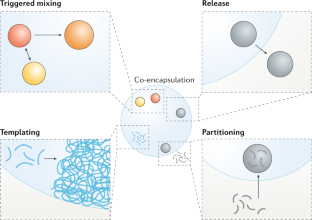
Similar content being viewed by others
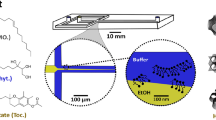
A microfluidic platform for the controlled synthesis of architecturally complex liquid crystalline nanoparticles
Article Open access 04 August 2023
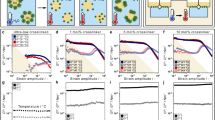
Interactions between interfaces dictate stimuli-responsive emulsion behaviour
Article Open access 23 October 2023
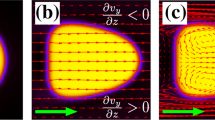
The vortex-driven dynamics of droplets within droplets
Article Open access 04 January 2021
References
- Brummer, R. & Godersky, S. Rheological studies to objectify sensations occurring when cosmetic emulsions are applied to the skin. Colloids Surf. A152, 89–94 (1999). CASGoogle Scholar
- Gilbert, L., Picard, C., Savary, G. & Grisel, M. Rheological and textural characterization of cosmetic emulsions containing natural and synthetic polymers: relationships between both data. Colloids Surf. A421, 150–163 (2013). CASGoogle Scholar
- O’Toole, J. T. Kinetics of emulsion polymerization. J. Appl. Polym. Sci.9, 1291–1297 (1965). Google Scholar
- Wang, L., Li, X., Zhang, G., Dong, J. & Eastoe, J. Oil-in-water nanoemulsions for pesticide formulations. J. Colloid Interface Sci.314, 230–235 (2007). CASGoogle Scholar
- Hainey, P., Huxham, I. M., Rowatt, B., Sherrington, D. C. & Tetley, L. Synthesis and ultrastructural studies of styrene-divinylbenzene polyhipe polymers. Macromolecules24, 117–121 (1991). CASGoogle Scholar
- Pileni, M.-P. The role of soft colloidal templates in controlling the size and shape of inorganic nanocrystals. Nat. Mater.2, 145–150 (2003). CASGoogle Scholar
- Bhumgara, Z. Polyhipe foam materials as filtration media. Filtr. Sep.32, 245–251 (1995). CASGoogle Scholar
- Helgeson, M. E. Colloidal behavior of nanoemulsions: interactions, structure, and rheology. Curr. Opin. Colloid Interface Sci.25, 39–50 (2016). CASGoogle Scholar
- Jaiswal, M., Dudhe, R. & Sharma, P. K. Nanoemulsion: an advanced mode of drug delivery system. 3 Biotech5, 123–127 (2015). Google Scholar
- Donalisio, M. et al. Acyclovir-loaded chitosan nanospheres from nano-emulsion templating for the topical treatment of herpesviruses infections. Pharmaceutics10, 46 (2018). Google Scholar
- Mahmood, T., Akhtar, N. & Manickam, S. Interfacial film stabilized W/O/W nano multiple emulsions loaded with green tea and lotus extracts: systematic characterization of physicochemical properties and shelf-storage stability. J. Nanobiotechnol.12, 20 (2014). Google Scholar
- Datta, S. S. et al. Double emulsion templated solid microcapsules: mechanics and controlled release. Adv. Mater.26, 2205–2218 (2014). CASGoogle Scholar
- Silva, B. F. B., Rodríguez-Abreu, C. & Vilanova, N. Recent advances in multiple emulsions and their application as templates. Curr. Opin. Colloid Interface Sci.25, 98–108 (2016). CASGoogle Scholar
- Abate, A. R. & Weitz, D. A. High-order multiple emulsions formed in poly(dimethylsiloxane) microfluidics. Small5, 2030–2032 (2009). CASGoogle Scholar
- Allouche, J., Tyrode, E., Sadtler, V., Choplin, L. & Salager, J.-L. Single- and two-step emulsification to prepare a persistent multiple emulsion with a surfactant–polymer mixture. Ind. Eng. Chem. Res.42, 3982–3988 (2003). CASGoogle Scholar
- Kim, S., Kim, K. & Choi, S. Q. Controllable one-step double emulsion formation via phase inversion. Soft Matter14, 1094–1099 (2018). CASGoogle Scholar
- Min, J.-Y., Ahn, S.-I., Lee, Y.-K., Kwak, H.-S. & Chang, Y. H. Optimized conditions to produce water-in-oil-in-water nanoemulsion and spray-dried nanocapsule of red ginseng extract. Food Science Technol.38, 485–492 (2018). Google Scholar
- Mason, T. G., Graves, S. M., Wilking, J. N. & Lin, M. Y. Extreme emulsification: formation and structure of nanoemulsions. Condens. Matter Phys.9, 193–199 (2006). Google Scholar
- McClements, D. J. & Rao, J. Food-grade nanoemulsions: formulation, fabrication, properties, performance, biological fate, and potential toxicity. Crit. Rev. Food Sci. Nutr.51, 285–330 (2011). CASGoogle Scholar
- Mason, T. G., Wilking, J. N., Meleson, K., Chang, C. B. & Graves, S. M. Nanoemulsions: formation, structure, and physical properties. J. Phys. Condens. Matter18, R635–R666 (2006). CASGoogle Scholar
- Bilati, U., Allémann, E. & Doelker, E. Sonication parameters for the preparation of biodegradable nanocapsules of controlled size by the double emulsion method. Pharm. Dev. Technol.8, 1–9 (2003). CASGoogle Scholar
- Koroleva, M. Y. & Yurtov, E. V. Nanoemulsions: the properties, methods of preparation and promising applications. Russ. Chem. Rev.81, 21–43 (2012). CASGoogle Scholar
- Solans, C. & Solé, I. Nano-emulsions: formation by low-energy methods. Curr. Opin. Colloid Interface Sci.17, 246–254 (2012). CASGoogle Scholar
- Forgiarini, A., Esquena, J., González, C. & Solans, C. Formation of nano-emulsions by low-energy emulsification methods at constant temperature. Langmuir17, 2076–2083 (2001). CASGoogle Scholar
- Anton, N. & Vandamme, T. F. The universality of low-energy nano-emulsification. Int. J. Pharm.377, 142–147 (2009). An adept description of the single, surfactant-driven mechanism through which spontaneous emulsification occurs, regardless of concentration or temperature driving force. CASGoogle Scholar
- Gupta, A., Badruddoza, A. Z. M. & Doyle, P. S. A general route for nanoemulsion synthesis using low-energy methods at constant temperature. Langmuir33, 7118–7123 (2017). CASGoogle Scholar
- Izquierdo, P. et al. Formation and stability of nano-emulsions prepared using the phase inversion temperature method. Langmuir18, 26–30 (2002). CASGoogle Scholar
- Leitner, S., Solans, C., García-Celma, M. J. & Calderó, G. Low-energy nano-emulsification approach as a simple strategy to prepare positively charged ethylcellulose nanoparticles. Carbohydr. Polym.205, 117–124 (2019). CASGoogle Scholar
- Yang, Y., Marshall-Breton, C., Leser, M. E., Sher, A. A. & McClements, D. J. Fabrication of ultrafine edible emulsions: comparison of high-energy and low-energy homogenization methods. Food Hydrocoll.29, 398–406 (2012). CASGoogle Scholar
- McClements, D. J. Food Emulsions: Principles, Practices, and Techniques 2nd edn (CRC, 2005).
- Para, G., Jarek, E., Warszyński, P. & Adamczyk, Z. Effect of electrolytes on surface tension of ionic surfactant solutions. Colloids Surf. A222, 213–222 (2003). CASGoogle Scholar
- Sainis, S. K., Germain, V., Mejean, C. O. & Dufresne, E. R. Electrostatic interactions of colloidal particles in nonpolar solvents: role of surface chemistry and charge control agents. Langmuir24, 1160–1164 (2008). CASGoogle Scholar
- Espinosa, C. E., Guo, Q., Singh, V. & Behrens, S. H. Particle charging and charge screening in nonpolar dispersions with nonionic surfactants. Langmuir26, 16941–16948 (2010). CASGoogle Scholar
- Tadros, T. F. Applied Surfactants: Principles and Applications (Wiley-VCH, 2005).
- Luan, F. et al. Prediction of hydrophile–lipophile balance values of anionic surfactants using a quantitative structure–property relationship. J. Colloid Interface Sci.336, 773–779 (2009). CASGoogle Scholar
- Florence, A. T. & Whitehill, D. The formulation and stability of multiple emulsions. Int. J. Pharm.11, 277–308 (1982). CASGoogle Scholar
- Pal, R. Multiple O/W/O emulsion rheology. Langmuir12, 2220–2225 (1996). CASGoogle Scholar
- Carlotti, M. E., Gallarate, M., Sapino, S., Ugazio, E. & Morel, S. W/O/W multiple emulsions for dermatological and cosmetic use, obtained with ethylene oxide free emulsifiers. J. Dispers. Sci. Technol.26, 183–192 (2005). CASGoogle Scholar
- Morais, J. M., Santos, O. D. H., Nunes, J. R. L., Zanatta, C. F. & Rocha-Filho, P. A. W/O/W multiple emulsions obtained by one-step emulsification method and evaluation of the involved variables. J. Dispers. Sci. Technol.29, 63–69 (2008). CASGoogle Scholar
- Fryd, M. M. & Mason, T. G. Advanced nanoemulsions. Annu. Rev. Phys. Chem.63, 493–518 (2012). CASGoogle Scholar
- Pawar, A. B., Caggioni, M., Ergun, R., Hartel, R. W. & Spicer, P. T. Arrested coalescence in Pickering emulsions. Soft Matter7, 7710–7716 (2011). CASGoogle Scholar
- Chevalier, Y. & Bolzinger, M.-A. Emulsions stabilized with solid nanoparticles: Pickering emulsions. Colloids Surf. A439, 23–34 (2013). CASGoogle Scholar
- Vignati, E., Piazza, R. & Lockhart, T. P. Pickering emulsions: interfacial tension, colloidal layer morphology, and trapped-particle motion. Langmuir19, 6650–6656 (2003). CASGoogle Scholar
- Lee, Y.-T. et al. Ultrasound-based formation of nano-Pickering emulsions investigated via in-situ SAXS. J. Colloid Interface Sci.536, 281–290 (2019). CASGoogle Scholar
- Gañán-Calvo, A. M., González-Prieto, R., Riesco-Chueca, P., Herrada, M. A. & Flores-Mosquera, M. Focusing capillary jets close to the continuum limit. Nat. Phys.3, 737–742 (2007). Google Scholar
- Saleem, R. & Ahmad, R. Effect of ultrasonication on secondary structure and heat induced gelation of chicken myofibrils. J. Food Sci. Technol.53, 3340–3348 (2016). CASGoogle Scholar
- Zhang, M. et al. Controlling complex nanoemulsion morphology using asymmetric cosurfactants for the preparation of polymer nanocapsules. Langmuir34, 978–990 (2018). CASGoogle Scholar
- Helfrich, W. Elastic properties of lipid bilayers: theory and possible experiments. Z. Naturforsch. C28, 693–703 (1973). CASGoogle Scholar
- Malo de Molina, P., Zhang, M., Bayles, A. V. & Helgeson, M. E. Oil-in-water-in-oil multinanoemulsions for templating complex nanoparticles. Nano Lett.16, 7325–7332 (2016). CASGoogle Scholar
- Hanson, J. A. et al. Nanoscale double emulsions stabilized by single-component block copolypeptides. Nature455, 85–88 (2008). This paper demonstrates the use of asymmetric co-surfactants to produce metastable core–shell double nanoemulsions. CASGoogle Scholar
- Fryd, M. M. & Mason, T. G. Cerberus nanoemulsions produced by multidroplet flow-induced fusion. Langmuir29, 15787–15793 (2013). This paper demonstrates the ability to form high-order structures by increasing the number of immiscible phases within dispersed droplets. CASGoogle Scholar
- Patel, S. K., Zhang, Y., Pollock, J. A. & Janjic, J. M. Cyclooxgenase-2 inhibiting perfluoropoly (ethylene glycol) ether theranostic nanoemulsions—in vitro study. PLOS ONE8, e55802 (2013). This paper highlights the development of a triphasic fluorocarbon-in-hydrocarbon-in-water core–shell double-nanoemulsion system and demonstrates its use for dual-mode imaging in vitro. CASGoogle Scholar
- Patel, S. K., Patrick, M. J., Pollock, J. A. & Janjic, J. M. Two-color fluorescent (near-infrared and visible) triphasic perfluorocarbon nanoemulsions. J. Biomed. Opt.18, 101312 (2013). Google Scholar
- Wu, S., Hung, Y. & Mou, C. Compartmentalized hollow silica nanospheres templated from nanoemulsions. Chem. Mater.25, 352–364 (2013). This paper highlights the use of interfacial silica polymerization to form nested double nanoemulsions for the potential application of co-encapsulation of water-soluble and oil-soluble drugs. CASGoogle Scholar
- van der Graaf, S., Schroën, C. G. P. H. & Boom, R. M. Preparation of double emulsions by membrane emulsification: a review. J. Membr. Sci.251, 7–15 (2005). Google Scholar
- Zambaux, M. F. et al. Influence of experimental parameters on the characteristics of poly(lactic acid) nanoparticles prepared by a double emulsion method. J. Control. Rel.50, 31–40 (1998). CASGoogle Scholar
- Tang, S. Y., Sivakumar, M. & Nashiru, B. Impact of osmotic pressure and gelling in the generation of highly stable single core water-in-oil-in-water (W/O/W) nano multiple emulsions of aspirin assisted by two-stage ultrasonic cavitational emulsification. Colloids Surf. B102, 653–658 (2013). CASGoogle Scholar
- Tang, S. Y., Sivakumar, M., Ng, A. M. & Shridharan, P. Anti-inflammatory and analgesic activity of novel oral aspirin-loaded nanoemulsion and nano multiple emulsion formulations generated using ultrasound cavitation. Int. J. Pharm.430, 299–306 (2012). CASGoogle Scholar
- Zhang, M. et al. Synthesis of oil-laden poly(ethylene glycol) diacrylate hydrogel nanocapsules from double nanoemulsions. Langmuir33, 6116–6126 (2017). This work demonstrates the technique of sequential emulsification and one of the resulting applications enabled by core–shell nanoemulsions — templating of oil-laden hydrogel nanocapsules. CASGoogle Scholar
- Nisisako, T., Okushima, S. & Torii, T. Controlled formulation of monodisperse double emulsions in a multiple-phase microfluidic system. Soft Matter1, 23–27 (2005). CASGoogle Scholar
- Chu, L.-Y., Utada, A. S., Shah, R. K., Kim, J. W. & Weitz, D. A. Controllable monodisperse multiple emulsions. Angew. Chem. Int. Ed.46, 8970–8974 (2007). CASGoogle Scholar
- Zhang, M.-Y., Zhao, H., Xu, J.-H. & Luo, G.-S. Controlled coalescence of two immiscible droplets for Janus emulsions in a microfluidic device. RSC Adv.5, 32768–32774 (2015). CASGoogle Scholar
- Park, J., Forster, J. D. & Dufresne, E. R. High-yield synthesis of monodisperse dumbbell-shaped polymer nanoparticles. J. Am. Chem. Soc.132, 5960–5961 (2010). CASGoogle Scholar
- Li, J. et al. A dewetting route to grow heterostructured nanoparticles based on thin film heterojunctions. Nanoscale7, 19977–19984 (2015). CASGoogle Scholar
- Lu, Y. et al. Asymmetric dimers can be formed by dewetting half-shells of gold deposited on the surfaces of spherical oxide colloids. J. Am. Chem. Soc.125, 12724–12725 (2003). CASGoogle Scholar
- Oh, C., Park, J., Shin, S. & Oh, S. O/W/O multiple emulsions via one-step emulsification process. J. Dispers. Sci. Technol.25, 53–62 (2004). CASGoogle Scholar
- Galindo-Alvarez, J., Sadtler, V., Choplin, L. & Salager, J.-L. Viscous oil emulsification by catastrophic phase inversion: influence of oil viscosity and process conditions. Ind. Eng. Chem. Res.50, 5575–5583 (2011). CASGoogle Scholar
- Liu, Y., Carter, E. L., Gordon, G. V., Feng, Q. J. & Friberg, S. E. An investigation into the relationship between catastrophic inversion and emulsion phase behaviors. Colloids Surf. A399, 25–34 (2012). CASGoogle Scholar
- Sigward, E. et al. Formulation and cytotoxicity evaluation of new self-emulsifying multiple W/O/W nanoemulsions. Int. J. Nanomed.8, 611–625 (2013). Google Scholar
- Shakeel, F., Haq, N., Al-Dhfyan, A., Alanazi, F. K. & Alsarra, I. A. Double w/o/w nanoemulsion of 5-fluorouracil for self-nanoemulsifying drug delivery system. J. Mol. Liq.200, 183–190 (2014). CASGoogle Scholar
- Sigward, E. et al. Preparation and evaluation of multiple nanoemulsions containing gadolinium (III) chelate as a potential magnetic resonance imaging (MRI) contrast agent. Pharm. Res.32, 2983–2994 (2015). CASGoogle Scholar
- Pangeni, R., Choi, S. W., Jeon, O.-C., Byun, Y. & Park, J. W. Multiple nanoemulsion system for an oral combinational delivery of oxaliplatin and 5-fluorouracil: preparation and in vivo evaluation. Int. J. Nanomed.11, 6379–6399 (2016). CASGoogle Scholar
- Ding, S. et al. A new method for the formulation of double nanoemulsions. Soft Matter13, 1660–1669 (2017). W/O/W double nanoemulsions are produced using an emulsification process involving a primary step of high-pressure homogenization to generate a W/O nanoemulsion, followed by spontaneous emulsification of the W/O nanoemulsion in water to stabilize W/O/W core–shell droplets. CASGoogle Scholar
- Lee, H. S., Morrison, E. D., Frethem, C. D., Zasadzinski, J. A. & McCormick, A. V. Cryogenic electron microscopy study of nanoemulsion formation from microemulsions. Langmuir30, 10826–10833 (2014). CASGoogle Scholar
- Lee, H. S., Morrison, E. D., Zhang, Q. & McCormick, A. V. Cryogenic transmission electron microscopy study: preparation of vesicular dispersions by quenching microemulsions. J. Microsc.263, 293–299 (2016). This study describes the technique of temperature-induced and concentration-induced microemulsion phase inversion to form core–shell and nested double nanoemulsions. CASGoogle Scholar
- Zhao, Y., Zhang, J., Wang, Q., Li, J. & Han, B. Water-in-oil-in-water double nanoemulsion induced by CO2. Phys. Chem. Chem. Phys.13, 684–689 (2011). CASGoogle Scholar
- Tiarks, F., Landfester, K. & Antonietti, M. Preparation of polymeric nanocapsules by miniemulsion polymerization. Langmuir17, 908–918 (2001). The demonstration that core–shell and lens-type droplets arise through dewetting of a monomer solution from polymerizing particles during nanoparticle synthesis. CASGoogle Scholar
- Grundy, L. S. et al. Rapid production of internally structured colloids by flash nanoprecipitation of block copolymer blends. ACS Nano12, 4660–4668 (2018). CASGoogle Scholar
- Lin, T. J. Low-energy emulsification I: Principles and applications. J. Soc. Cosmet. Chem.29, 117–125 (1978). Google Scholar
- Komaiko, J. S. & McClements, D. J. Optimization of isothermal low-energy nanoemulsion formation: hydrocarbon oil, non-ionic surfactant, and water systems. J. Colloid Interface Sci.425, 59–66 (2014). CASGoogle Scholar
- Komaiko, J. S. & McClements, D. J. Low-energy formation of edible nanoemulsions by spontaneous emulsification: factors influencing particle size. J. Food Eng.146, 122–128 (2015). CASGoogle Scholar
- Komaiko, J. S. & McClements, D. J. Formation of food-grade nanoemulsions using low-energy preparation methods: a review of available methods. Compr. Rev. Food Sci. Food Saf.15, 331–352 (2016). CASGoogle Scholar
- Provencher, S. W. A constrained regularization method for inverting data represented by linear algebraic or integral equations. Comput. Phys. Commun.27, 213–227 (1982). Google Scholar
- Thomas, D. G. Transport characteristics of suspension: VIII. A note on the viscosity of Newtonian suspensions of uniform spherical particles. J. Colloid Sci.20, 267–277 (1965). CASGoogle Scholar
- Tadros, T. F., Izquierdo, P., Esquena, J. & Solans, C. Formation and stability of nano-emulsions. Adv. Colloid Interface Sci.108–109, 303–318 (2004). Google Scholar
- Gupta, A., Eral, H. B., Hatton, T. A. & Doyle, P. S. Nanoemulsions: formation, properties and applications. Soft Matter12, 2826–2841 (2016). CASGoogle Scholar
- McClements, D. J. Nanoemulsions versus microemulsions: terminology, differences, and similarities. Soft Matter8, 1719–1729 (2012). CASGoogle Scholar
- Matalanis, A., Jones, O. G. & McClements, D. J. Structured biopolymer-based delivery systems for encapsulation, protection, and release of lipophilic compounds. Food Hydrocoll.25, 1865–1880 (2011). CASGoogle Scholar
- Qian, J. & Law, C. K. Regimes of coalescence and separation in droplet collision. J. Fluid Mech.331, 59–80 (1997). CASGoogle Scholar
- Leal, L. G. Flow induced coalescence of drops in a viscous fluid. Phys. Fluids16, 1833–1851 (2004). CASGoogle Scholar
- Baldessari, F. & Leal, L. G. Effect of overall drop deformation on flow-induced coalescence at low capillary numbers. Phys. Fluids18, 013602 (2006). Google Scholar
- Rother, M. A. & Davis, R. H. The effect of slight deformation on droplet coalescence in linear flows. Phys. Fluids13, 1178–1190 (2001). CASGoogle Scholar
- Voorhees, P. W. The theory of Ostwald ripening. J. Stat. Phys.38, 231–252 (1985). Google Scholar
- Taylor, P. Ostwald ripening in emulsions. Adv. Colloid Interface Sci.75, 107–163 (1998). CASGoogle Scholar
- Lifshitz, I. M. & Slyozov, V. V. The kinetics of precipitation from supersaturated solid solutions. J. Phys. Chem. Solids19, 35–50 (1961). Google Scholar
- Roger, K., Olsson, U., Schweins, R. & Cabane, B. Emulsion ripening through molecular exchange at droplet contacts. Angew. Chem. Int. Ed.54, 1452–1455 (2015). CASGoogle Scholar
- Ficheux, M.-F., Bonakdar, L., Leal-Calderon, F. & Bibette, J. Some stability criteria for double emulsions. Langmuir14, 2702–2706 (1998). CASGoogle Scholar
- Pays, K., Giermanska-Kahn, J., Pouligny, B., Bibette, J. & Leal-Calderon, F. Double emulsions: How does release occur? J. Control. Rel.79, 193–205 (2002). CASGoogle Scholar
- Pays, K., Giermanska-Kahn, J., Pouligny, B., Bibette, J. & Leal-Calderon, F. Coalescence in surfactant-stabilized double emulsions. Langmuir17, 7758–7769 (2001). CASGoogle Scholar
- Jiao, J., Rhodes, D. G. & Burgess, D. J. Multiple emulsion stability: pressure balance and interfacial film strength. J. Colloid Interface Sci.250, 444–450 (2002). CASGoogle Scholar
- Schmidts, T., Dobler, D., Nissing, C. & Runkel, F. Influence of hydrophilic surfactants on the properties of multiple W/O/W emulsions. J. Colloid Interface Sci.338, 184–192 (2009). CASGoogle Scholar
- Zeeb, B., Saberi, A. H., Weiss, J. & McClements, D. J. Retention and release of oil-in-water emulsions from filled hydrogel beads composed of calcium alginate: impact of emulsifier type and pH. Soft Matter11, 2228–2236 (2015). CASGoogle Scholar
- Muschiolik, G. Multiple emulsions for food use. Curr. Opin. Colloid Interface Sci.12, 213–220 (2007). CASGoogle Scholar
- Sapei, L., Naqvi, M. A. & Rousseau, D. Stability and release properties of double emulsions for food applications. Food Hydrocoll.27, 316–323 (2012). CASGoogle Scholar
- Kawasaki, J., Kosuge, H., Egashira, R. & Asawa, T. Mechanical entrainment in W/O/W emulsion liquid membrane. Sep. Sci. Technol.44, 151–168 (2009). CASGoogle Scholar
- Chakraborty, M. & Bart, H.-J. Emulsion liquid membranes: role of internal droplet size distribution on toluene/n-heptane separation. Colloids Surf. A272, 15–21 (2006). CASGoogle Scholar
- Gupta, S., Chakraborty, M. & Murthy, Z. V. P. Removal of mercury by emulsion liquid membranes: studies on emulsion stability and scale up. J. Dispers. Sci. Technol.34, 1733–1741 (2013). CASGoogle Scholar
- Hamidi, M., Azadi, A. & Rafiei, P. Hydrogel nanoparticles in drug delivery. Adv. Drug. Deliv. Rev.60, 1638–1649 (2008). CASGoogle Scholar
- Gawande, M. B. et al. Core–shell nanoparticles: synthesis and applications in catalysis and electrocatalysis. Chem. Soc. Rev.44, 7540–7590 (2015). CASGoogle Scholar
- Schleich, N. et al. Dual anticancer drug/superparamagnetic iron oxide-loaded PLGA-based nanoparticles for cancer therapy and magnetic resonance imaging. Int. J. Pharm.447, 94–101 (2013). CASGoogle Scholar
- Matsushita, H. et al. Multifunctional core–shell silica nanoparticles for highly sensitive 19 F magnetic resonance imaging. Angew. Chem. Int. Ed.53, 1008–1011 (2014). CASGoogle Scholar
- Schwarz, J. C. et al. Optimisation of multiple W/O/W nanoemulsions for dermal delivery of aciclovir. Int. J. Pharm.435, 69–75 (2012). CASGoogle Scholar
- Helgeson, M. E., Moran, S. E., An, H. Z. & Doyle, P. S. Mesoporous organohydrogels from thermogelling photocrosslinkable nanoemulsions. Nat. Mater.11, 344–352 (2012). CASGoogle Scholar
- An, H. Z., Helgeson, M. E. & Doyle, P. S. Nanoemulsion composite microgels for orthogonal encapsulation and release. Adv. Mater.24, 3838–3844 (2012). CASGoogle Scholar
- Anton, N., Mojzisova, H., Porcher, E., Benoit, J. & Saulnier, P. Reverse micelle-loaded lipid nano-emulsions: new technology for nano-encapsulation of hydrophilic materials. Int. J. Pharm.398, 204–209 (2010). CASGoogle Scholar
- Vilanova, N., Kolen’ko, Y. V., Solans, C. & Rodríguez-Abreu, C. Multiple emulsions as soft templates for the synthesis of multifunctional silicone porous particles. J. Colloid Interface Sci.437, 235–243 (2015). CASGoogle Scholar
- Arriaga, L. R., Amstad, E. & Weitz, D. A. Scalable single-step microfluidic production of single-core double emulsions with ultra-thin shells. Lab Chip15, 3335–3340 (2015). CASGoogle Scholar
- Abbaspourrad, A., Carroll, N. J., Kim, S.-H. & Weitz, D. A. Polymer microcapsules with programmable active release. J. Am. Chem. Soc.135, 7744–7750 (2013). CASGoogle Scholar
- Kim, S.-H. et al. Formation of polymersomes with double bilayers templated by quadruple emulsions. Lab Chip13, 1351–1356 (2013). CASGoogle Scholar
- Hwang, T.-L., Fang, C.-L., Chen, C.-H. & Fang, J.-Y. Permeation enhancer-containing water-in-oil nanoemulsions as carriers for intravesical cisplatin delivery. Pharm. Res.26, 2314–2323 (2009). CASGoogle Scholar
- de Jonge, N. & Ross, F. M. Electron microscopy of specimens in liquid. Nat. Nanotechnol.6, 695–704 (2011). Google Scholar
- Evans, J. E., Jungjohann, K. L., Browning, N. D. & Arslan, I. Controlled growth of nanoparticles from solution with in situ liquid transmission electron microscopy. Nano Lett.11, 2809–2813 (2011). CASGoogle Scholar
- Yuk, J. M. et al. High-resolution EM of colloidal nanocrystal growth using graphene liquid cells. Science336, 61–64 (2012). CASGoogle Scholar
- Shum, H. C. et al. Droplet microfluidics for fabrication of non-spherical particles. Macromol. Rapid Commun.31, 108–118 (2010). CASGoogle Scholar
- Glotzer, S. C. & Solomon, M. J. Anisotropy of building blocks and their assembly into complex structures. Nat. Mater.6, 557–562 (2007). Google Scholar
Acknowledgements
T.P. was financially supported by the California Research Alliance by BASF. T.S. and M.E.H. were financially supported by the National Science Foundation under award no. CBET 1351371.